Welcome back to another addition of the Simply Complex Blog! This time around is going to be an extension of one my previous posts, The Physics of Athletic Performance: A Beginner's Guide to The Impulse-Momentum Relationship. There, we introduced the general concept of the impulse-moment relationship and how the laws of Newtonian physics play a instrumental role in understanding how we assess movement in athletics and human locomotion as a whole. Well now I want to go a step further and look at one of the specific scenarios in which we use impulse to measure and analyze performance: vertical jumps.
Jump Testing
As a basic principle of training, we're usually looking for markers of progress. I mean, speaking realistically, we can't honestly measure our success as a performance coach/trainer on our athletes' success in their sport. Well, not directly. Our mission should be to improve the physical systems they use within their athletic endeavors so that they have the necessary tools to execute the specific skills they need. Along with the resiliency to accumulate the reps necessary to master those skills. So, our focus should be on measuring changes in those physical capacities to understand the impacts of our training. While there is a whole other conversation to be had about what could and should be measured, we're going to focus on the topic of impulse and how our athlete’s express force.
With most ground-based power sports, the athlete’s ability to apply force rapidly to the ground is viewed as an essential training focus because it is the universal application of creating impulse with the objective of moving their center of mass. Simply put, gravity is always working on us. And if we want to move, we need to push into the ground to create ground reaction forces (GRF). Those forces are a direct reflection of the force we create with our mass and our muscles. That force works against our mass [impulse] to create a change in our momentum in the direction of that force.
While this is a drawn-out explanation of common knowledge, I do think it's important to reflect on because of its importance to the topic. It's the foundation of all athletic performance training principles. In some form of fashion, we are looking to create an adaptation that leads to our athletes' ability to produce more force (or produce force faster) in such a way that it improves their GRF and resulting momentum outcomes. But with this in mind, are we testing our outcomes with the same perspective?
Vertical Jump Assessments
One of the most common ways we test for these types of training adaptations is with jump testing. And it makes total sense! What better way to assess an athlete’s ability to oppose gravity than to see how high they can get?! Going back to our guy Newtown's laws... we know that for an object to move upwards and opposed to gravity, it must have an impulse in the opposite direction that results in a change in its acceleration and resulting momentum. Well, if that impulse is great enough, there will be such large change in acceleration that the mass's momentum will carry on until the negative acceleration of gravity (decreasing the masses acceleration) has slowed it down enough to change its direction back downward. This is the thought process behind propulsion and flight. Big impulse, big flight; Bigger impulse, bigger flight.
This is what makes jump testing such a great tool. We can measure if the athlete has gained the ability to create higher flight. And we can measure it multiple ways. But there are some caveats with this ideology. It's important to understand, like with all test - retest comparisons, we need to have an idea of what meaningful change looks like. We need to be able to understand what amount of change significant and not just normal variation. Without this, we really can't achieve our goal of measuring the effect of our training. Keeping this in mind, it plays a big part in understanding that all jump tests are not created equal. The way we measure the jump makes a difference in our results.
Measurement Methods
Displacement
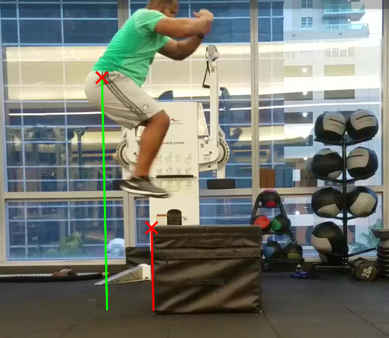
The most common method of calculating jump height is by measuring displacement. It's the most rudimentary and was once the most easily reproducible way to test a jump. You start "here" and I mark the distance you traveled from the jump. With vertical jump tests, this is done with a standing reach, followed by marking the highest point their hand reaches during the flight of the jump. Or in a broad jump, we can measure the distance from their toes at takeoff to their heels at landing. Each gives us a displacement that is approximately a result of the impulse they created.
Flight Time
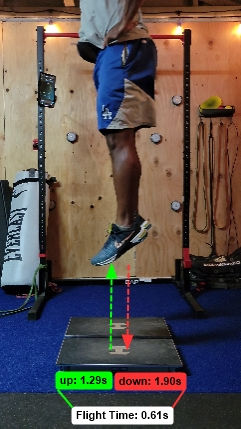
As I just mentioned, displacement used to be the easiest way to reproduce jump results. But over the last couple of decades, flight Time has become an even easier and readily available method due to the lowering costs of technology. More advanced devices, like contact mats, allow the jump height to be calculated by flight time. But more recently, jump heights are being collected with ease by one of our most common tools, cell phones. Whether you're using an app made for measuring jump height, or just watching the recording time elapse in slow motion video you captured, the quality of the modern cell phone camera has made this a realistic tool for testing jump height.
Time in air can be used to calculate jump height because of the constant negative acceleration of gravity. Since gravity is always working to accelerate a mass downward at 9.81 m/s^2, it takes as much time to slow the mass down on its way up, as it does to bring the mass back down to earth. This means that we know the distance that could be covered in ½ the amount of the total flight time. We can easily measure the jump height (jh) using the formula below. Where g = 9.81 (gravity), and t = the flight time recorded.
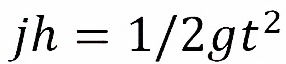
Impulse-Momentum
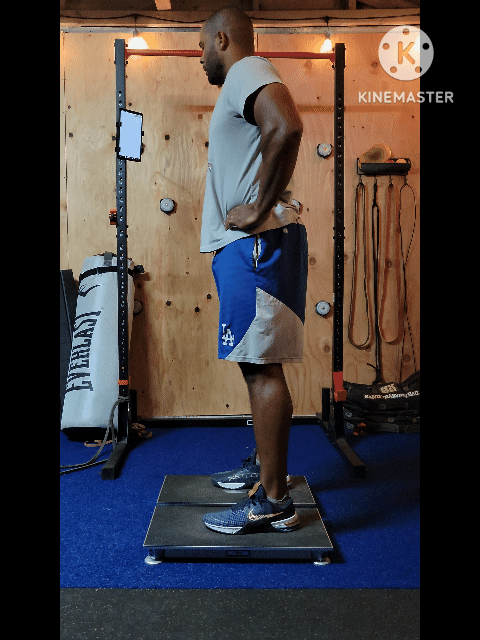
Another benefit of the modern-day tech boom is the relatively low cost of hardware and software. Devices like stain gauges, which measure tensile force, are a fraction of their cost 20 years ago. That, along with the many advancements in software development, has made the world of commercial force plates a real option for many more coaches and sports scientists. With force Platforms [plates], we can directly masure the ground reaction forces. That means we can calculate our changes in impulse, and momentum, in real time. Using our understanding of the impulse-momentum relationship, we can calculate the velocity of center of mass at takeoff [when the athlete leaves the ground] and thus know the height the mass will travel.
How Do They Differ?
Jump testing might seem straightforward, but it's not a one-size-fits-all solution. The reliability and validity of our testing methods matter, as they dictate the confidence we can have in the results and the level of variability we're willing to accept.
Consider this: it's fine if your weather app is off by a few degrees ( +/- 3 degrees). It wont really change your mind on if you need a coat. But when it comes to finding a stud in the wall, plus or minus 3 inches could be the difference in repairing your wall and buying a new TV.
Similarly, when assessing athletes, various factors influence jump height, and changes should be viewed in the context of these factors. Did the athlete's weight change? Is the surface consistent? Was the warm-up the same? But the most crucial question is whether the athlete's technique or movement strategy changed. Did their mobility or execution of the jump change?
These nuances can't be captured by displacement and flight time measurements, which introduce variability in jump assessments. We're interested in understanding an athlete's ability to generate impulse, and time plays a significant role. An athlete's strategy affects both the time to leave the ground and the force generated. Force plates offer a deeper comprehension of the "why" behind jump output changes and provide more reliable measurements, enhancing our confidence in the results compared to other methods.
Calculating Jump Metrics from Impulse
With all that said, let’s look at how we can actually see much more with GRFs than just jump height!
If we think back to the previous blog, we talked about how impulse has a direct relationship with momentum. And how momentum is, by definition, the kinetic energy of a mass in motion (its mass x it’s velocity). Well, this means that we can see changes in a mass’s velocity based on its impulse at a given point in time.
The same is true for a person standing on a force plate. Just standing on the platform allows us to know their mass at rest and creates a starting point to measure changes in momentum and velocity. In terms of measuring jump height, the velocity at takeoff is the key to calculating the jump height.
Like the equation used to measure jump height with contact time, the constant of gravity and time allow for us to use a ‘plug and play’ approach with these calculations. With the having the mass of the person, we know that any impulse greater than that will have a positive net impulse. That positive net impulse allows us to measure the change in the
momentum, since a change in momentum is equal to the force accumulated in that time.

With momentum being 'mass x velocity', we can calculate the jumper’s velocity at takeoff. Knowing that velocity allows us to use a similar equation to the flight time equation. In this case, we have velocity as the known variable to apply to the negative deceleration of gravity used to calculate the distance they will travel upward before their positive velocity is lowered to zero and they are returned down to earth. This scenario we use the inverse of the flight time integration and multiply gravity by 2, instead of dividing it in half. Here we square the takeoff velocity, divide it by 2 times gravity to calculate the jump height.

While the singular result of jump height is the same (and more accurate); we can use other equations to learn so much more. For example, with impulse we can identify the different phases of the jump strategy itself. We can measure the amount of depth during the descent of the unweighting phase. We can measure the lowest point of the center of mass during the braking phase, and the force produced there. This is commonly referred to as the amortization phase. We can also measure the differences in braking/decelerating forces from the propulsive/accelerating forces of the jumper.
Breaking Down the Countermovement Jump

The countermovement jump (the technical name for what we commonly call ‘vertical leap testing’ at the combines and pro days) consists of 3 distinct phases: unweighting, braking, and propulsive. And each of these phases can be identified simply by analyzing changes in impulse relative to system mass [body weight].
Unweighting
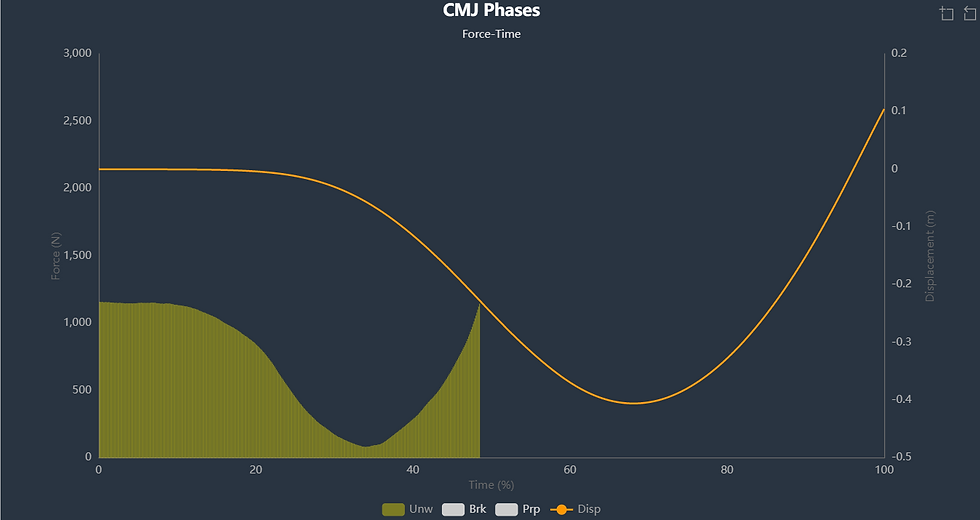
When the athlete starts their initial descent downwards, their impulse goes below their system mass. This means the athlete is allowing gravity to move them downward and create a negative velocity. Hence the name [unweighting]; because the athlete is “decreasing their resistance” to gravity and lessening their weight in an effort to use the negative velocity to create negative momentum. This negative momentum is useful in creating kinetic energy that can be stored and utilized by our elastic tendon and muscle fibers.
While the unweighting phase is the definition of ‘anti-climactic’. It does serve as the introduction to ‘the story’ of the jump. In this phase we can see if an athlete shows inhibition, or hesitancy, when starting their jump. Which is often the case when athletes are returning from lower limb injury.
Braking
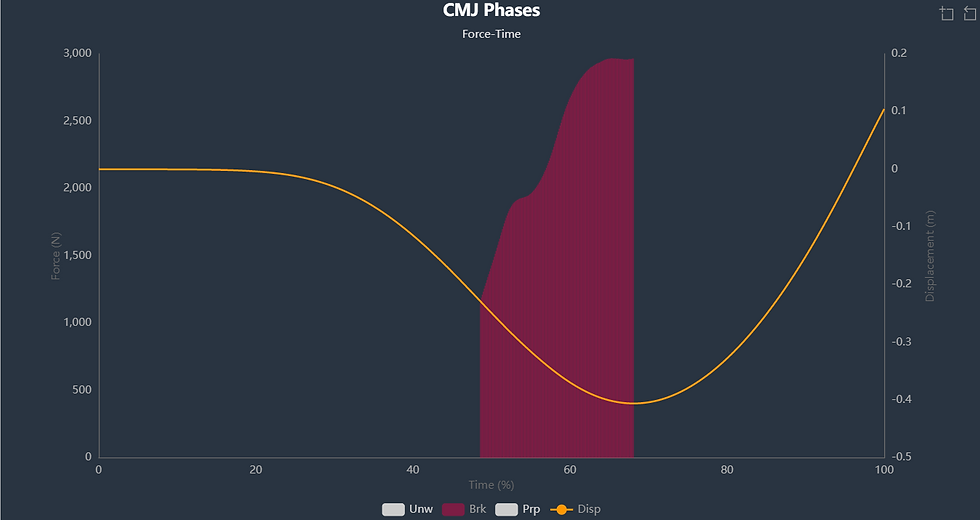
Obviously, the athlete wants to start creating force to change their path and start heading upwards. When the athlete starts to create force that reaches back to the impulse of their system weight, this is the beginning of the braking phase. The braking phase is often, and incorrectly, referred to as the eccentric phase. This is incorrect in the sense that the term eccentric refers to a type of muscular contraction. Some of the primary muscles involved in knee extension and plantar flexion are experiencing eccentric contractions. But the system is working to stop the descent of the center of mass.
The braking phase gives a wealth of information about the athlete. It gives insights into phase specific strength, power, and even the rate of force development. These phase specific metrics are what allows us to understand the athlete’s capacity for ballistic actions. The quality of the braking phase is a determining factor in how the athlete demonstrates reactive and dynamic strength.
Propulsive

The final and most important part of the jump is the propulsive phase. This phase starts at the point in which the athlete stops their downward movement [point of zero velocity], and uses the energy stored from the braking phase to continue to build impulse and create upward movement and momentum until that carries them off the ground [takeoff]. As I said earlier, the velocity at the point of takeoff determines the jump height.
But more than jump height, with the impulse we can see more intrinsic measures of athletic ability. Once again, we can measure peak and average force during this phase. We can see the rate at which the athlete produces force, and the resulting power produced. We can compare the forces and distribution of the propulsive phase to that of the braking phase. Comparing the phases allows us to understand the strategies specific to the athlete and create a sort of ‘signature’ that is specific to them. And more so, we can see if this signature changes from the effect of our training prescription.
Conclusion
With this type of information, we have a much more holistic view of how our training has altered the athlete’s expression of force. Instead of just looking at the outcome through a hazy lens of low confidence in the results, we can have much more clarity in how we influence the factors that the results are derived.
While this post was specific to analyzing impulse of the CMJ, there is much more to analyze about a countermovement jump. Jumps can be used in many ways, including talent identification, return to play, evaluation, and pre and post training phase comparisons. There are also other jump test variations that were developed to identify specific physical qualities that aren’t as well represented by the CMJ. In later posts we will look at further into how we can use the CMJ for assessing our athletes, as well as the use of the other tests and what they can do for us. Thanks for flying with us, and we hope you will choose Greenhouse Airlines again for your next journey in to sports science. ✈️
Comments